MSU scientist catches subatomic glimpse into 3-D structure of all matter
Over the past century, physicists have pieced together the basic building blocks of the universe like a giant jigsaw puzzle, one experiment at a time, inventing highly advanced instruments like Michigan State University’s National Superconducting Cyclotron Laboratory and Facility for Rare Isotope Beams to test their theories and dig deeper into subatomic world.
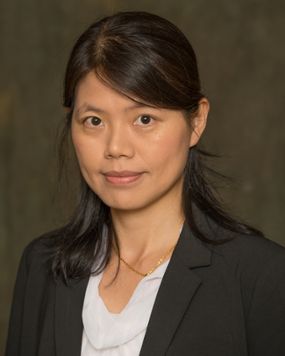
Research conducted by Huey-Wen Lin, an associate professor with joint appointments in the Department of Physics and Astronomy and the Department of Computational Mathematics, Science and Engineering in the MSU College of Natural Science, has just provided a major piece of the puzzle. For the first time, Lin used advanced calculations in lattice quantum chromodynamics, or lattice QCD, to directly measure the momentum of quarks inside the center of an atom and to generate three-dimensional images of the proton’s structure.
Her results were recently published in the journal Physical Review Letters.
“This research is one of the first lattice-QCD calculations able to describe the structure of the nucleon in terms of the momentum fraction carried by the quarks,” explained Lin, whose research uses high-performance computers to calculate the intricate interactions. “For most of the history of lattice QCD, it was impossible to make such a measurement, and we had to make do with more indirect measures. This work also provides the very first imaging of the proton’s 3D distributions from the Standard Model.”
The Standard Model, developed in the 1970s, describes all matter of the universe as made from a few fundamental particles, including quarks, gluons and electrons, and fundamental forces—strong, weak and electromagnetic. So far, the theory has been highly successful at explaining and predicting the outcome of particle physics experiments.
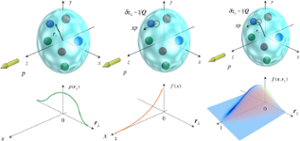
QCD aims to explain interactions between these fundamental particles and fundamental forces in the Standard Model. Lin’s research focuses on lattice QCD, a theory used to simulate those interactions at the tiniest scale, the femtoscale, using a lattice of points with trillions of possible parameters, or degrees of freedom.
“Considering physics at this tiny scale helps us understand how fundamental particles, quarks and gluons, make up the building blocks of all ordinary matter: nucleons—that is, protons and neutrons,” Lin said. “At the nuclear level, we can learn how the residual strong force binds these nucleons together into the nuclei that form the cores of all atoms, powers the stars and perhaps one day, fusion power plants.”
Particle accelerator laboratories all over the world have been devoted to searching for the last unknowns of the nucleon for more than half a century, but while our understanding of nucleon structure has improved over the past few decades, Lin says scientists still have a lot to learn about QCD.
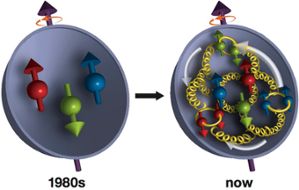
“Many QCD questions remain: What is the exact size of the proton? What gives the proton its mass? What's the origin of the proton's spin?” Lin explained. “One of the most intriguing ways to answer these questions is through the study of the nucleon’s three-dimensional structure, which we describe using functions such as generalized parton distributions, or GPDs.”
Utilizing a new method called large-momentum effective theory (LaMET), Lin’s calculations provided the first ever glimpse into the nucleon’s very structure via the GPDs.
“Using many years of computational resources at national supercomputer facilities, we can finally provide answers by studying the proton's three-dimensional structure, which has only been studied so far through models and assumptions,” Lin said. “We demonstrate LaMET really works by summing up the slices of the nucleon in our image in different ways to reproduce lattice calculations using older methods. This has never been done before and is the ultimate victory of such a method.”
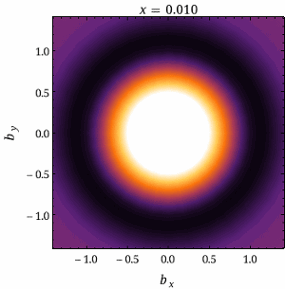
With this new three-dimensional information, scientists can create a kind of “CAT scan” of the proton and map out the probability of finding quarks at any given parameter within the nucleon—a new advance in this field of science.
In the future, elucidating these subatomic interactions may improve our understanding of objects at the astrophysical scale, such as the conditions in the center of neutron stars where incredible pressure and density could allow exotic forms of matter to exist.
“An understanding of how nuclear structure arises from fundamental physics may hold the key to the question why our universe seems fine-tuned for life—a mystery relevant to any carbon-based life forms,” Lin said. “Current and future generations of QCD experiments will continue helping us advance our knowledge of nucleon structure, but there are challenges ahead. Lattice QCD can help with the experimentally difficult to measure physics quantities, providing the missing clues in solving the remaining mystery of the proton.”
Banner image: Huey-Wen Lin’s ground-breaking research produced a “CAT scan” of the nucleon for the first time. The color represents the probability of finding a quark at a given “impact parameter” (labeled on the frame, b_x and b_y). The lighter color represents a higher probability of finding a quark. Credit: Huey-Wen Lin